Like clockwork
Why circadian rhythms matter
Features

The Earth takes approximately 24 hours to rotate on its axis, and all light-sensitive life on Earth has internal, biological clocks that anticipate and adapt to that daily cycle. Wake, work, eat, play, sleep; lather, rinse, repeat. From rose to rhinoceros, from mushroom to man, we all have a round-the-clock rhythm—scientifically known as the circadian rhythm, from the Latin circa diem, meaning ‘around the day.’
In recent years, the body of knowledge on circadian rhythm has grown dramatically. Scientists now understand that circadian rhythm regulates sleep patterns, and affects hormone release, metabolism, body temperature, and other functions. Biological clockwork that runs too fast or too slowly can result in irregular rhythms, which in turn can lead to a variety of health problems, including insomnia, mental illnesses, obesity, and diabetes. In fact, circadian rhythms affect everything from athletic performance—world records are more likely to be smashed in the afternoon than in the morning—to analytical agility.
It is clear today that the 24-hour nature of our Earthly existence is truly fundamental to our lives. This realization was signified by the fact that the Nobel Prize in Physiology or Medicine was awarded in 2017 to Jeffrey Hall, Michael Rosbash, and Michael Young for their discoveries of molecular mechanisms controlling the circadian rhythm. Prof. Gad Asher, a self-described ‘chronobiologist’ in the Department of Biomolecular Sciences who has made major insights in this area as well, anticipates that the Nobel Prize will inspire much-needed awareness among scientists of the impact of circadian rhythms on all aspects of science—especially in medicine and health.
“The importance of our bodies’ circadian clocks on every aspect of our physiology and behavior has always been anecdotal,” he says. “But now there’s growing appreciation of the molecular mechanisms involved—and the medical implications. It isn’t just what you eat, but also when you eat it; likewise, the timing of administering certain medications. It’s all about timing.”
Timing is everything
While scientists and laymen alike had appreciated the impact of daylight and darkness on people and plants for hundreds of years, it wasn’t until the 1970s that scientists began to understand the genetic mechanisms responsible for adapting the biological clocks of plants, animals, and humans to be in synchrony with the Earth’s revolutions.
In the 1970s, Caltech’s Prof. Seymour Benzer and his student Ronald Konopka first demonstrated that mutations in an unknown gene disrupted the circadian clock of fruit flies. They named this gene “period,” a scientific term related to frequency. Then, in 1984, Dr. Jeffrey Hall (a protégé of Benzer) and Dr. Michael Rosbash, working in close collaboration at Brandeis University in Boston, and, in parallel, Dr. Michael Young at the Rockefeller University in New York, succeeded in isolating the period gene, and went on to make key discoveries in this area.
The Brandeis duo discovered that PER, the protein encoded by the period gene, accumulated in all the body’s cells during the night and was degraded during the day, so its levels varied rhythmically over a 24-hour cycle. They then hypothesized that PER was in fact self-regulating: its own presence blocked the activity of the period gene. But how? How can a protein that is floating around in the cell affect DNA in the nucleus? The answer came in 1994, when Dr. Young discovered a second clock gene, which he dubbed “timeless” and which encoded the protein TIM. He showed that when TIM binds to PER, the dynamic duo is able to enter the nucleus where PER then acts to prevent the period gene from working.
By the mid-90s, it was clear that all these cellular events contributed to creating the self-regulating feedback loop at the heart of the circadian clock. The next question that arose was: How was it so precise—the biological equivalent of Greenwich Mean Time (GMT)? Dr. Young identified yet another clock gene, dubbed “doubletime”, that encodes the protein DBT which helps adjust PER and TIM levels over the course of the day. The discovery of these interactions established the fundamental principles underlying how biological clocks produce circadian rhythms and regulate their timing.
The master clock
With miniature, self-sustaining clocks in every cell, scientists wanted to know how everything is so perfectly coordinated. That is, where is a body’s master clock? It turns out that every creature has one, and in vertebrate animals, including humans, the master clock is a group of about 20,000 neurons that form a structure known as the suprachiasmatic nucleus (SCN)—a big name for a tiny part of the brain in the hypothalamus. The SCN receives input from the optic tract, which carries signals from the retina. Thus, the SCN has a direct, one-way input line from the eyes, which conveys information about how much light is visible—which, of course, varies periodically every 24 hours.
In this way, our master clocks are regulated by the sun. Sunlight-signals turn different genes in the SCN on and off, resetting our master clock, and by extension, our circadian rhythms. The SCN itself controls the production of a hormone, called melatonin, which induces sleepiness. When there is less light—as at night—the SCN induces the production of lots of melatonin, causing you to become drowsy. Thus, the mini-clocks throughout the body become part and parcel of the body’s overall sleep-wake cycle.
This light sensitivity explains why people get jet lag when they travel across time zones. That is, when there is a shift in the relationship between the behavioral rhythm and the light cycle, the behavioral rhythms must be re-set—a process that occurs within a few days of the shift, and as travelers well know, just in time to return home to get hit with jet lag again.
Important research is currently underway regarding the relationship between the immune system and circadian rhythms, with widespread implications. Some scientists have even suggested that due to the immune system’s own functional rhythm, it may be more effective to receive certain vaccines in the morning, rather than the afternoon. And the efficacy of timing the administration of certain pharmaceuticals according to circadian rhythms also could aid patients suffering from many diseases and disorders.
Moreover, researchers have advised that synchronizing our lives to a proper circadian rhythm can have a powerfully positive impact on our quality of life—like fine-tuning when we exercise or to try to conceive a baby, as Prof. Asher suggests. In time, he adds, we may be able to determine the optimal times for drug administration (with different clocks for different types of drugs), food intake, and even surgical procedures. “So many drugs interact with enzymes that are rhythmically expressed—the activity of a large fraction of the enzymes in our bodies follows circadian rhythms,” he says.
Prof. Gad Asher is supported by the Adelis Foundation, the European Research Council, and the Yeda-Sela Center for Basic Research.
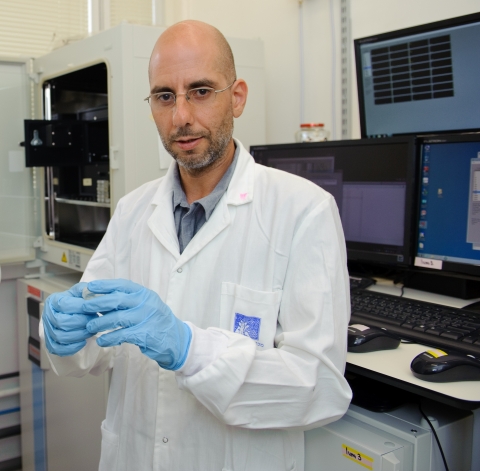
Prof. Gad Asher