Resolution revolution
Modern microscopy’s impact on science
Features

“Like you can’t have a car that can take the kids to schools on Friday and win the Grand Prix on Saturday, you can’t make a microscope that can do it all.”
– Dr. Eric Betzig, 2014 Nobel Laureate in Chemistry
Since their invention in the 1600s, microscopes have opened the window on human and plant biology, allowing scientists to marvel at things unavailable to the naked eye—and explore them in earnest. Developments have occurred over the last 400 years, but scientists agree that the last 15 years has seen nothing short of a “resolution revolution” with dramatic advancements that have ignited a new era in scientific research, allowing scientists to peer into the particulars of life sciences in unprecedented ways.
The secret lives of cells, in health and disease, are increasingly open to view.
We now have the ability to zoom in on the minutest workings of the molecules of life in a more naturalistic setting—and to do so at speeds that would have astonished researchers a mere 20 years ago. Weizmann Institute Prof. Ada Yonath’s painstaking work to understand protein structure, beginning in the 1980s—a herculean, Nobel Prizewinning effort of perseverance that lasted almost two decades—may now be accomplished in a comparative blink of an eye.
“Every new advance is contributing a puzzle piece of information to help illuminate biology, insights that will translate into meaningful results in human health.”
The earliest microscopes utilized light to image the sample, and thus were called optical microscopes. In the early 20th century, electron microscopy entered the scene, utilizing electrons to generate the images. Even today, the world of microscopy can be divided into two hemispheres: light (optical) microscopy (LM) and electron microscopy (EM). Although electron microscopes have long outpaced the resolution of their optical cousins, light microscopes have experienced a resolution revolution over the past decade and a half, thanks to advances in applying fluorescence to microscopy. At the same time, EM has experienced its own resolution revolution thanks to advances in single-particle imaging, allowing for near-atomic-level resolution of biological samples.
The Weizmann Institute of Science has capitalized on these parallel bouts of imaging innovation through active recruitment of talented microscopy experts, acquisition of state-of-the-art microscopy equipment, and the infrastructure needed to support mighty explorations on the smallest of scales—improving the power and quality of Weizmann research on cancer, stem cells, bones, muscles, tissues, and more.
Dr. Yoseph Addadi, scientific head of the de Picciotto-Lesser Cancer Cell Observatory in Memory of Wolfgang and Ruth Lesser in the MICC.
Both LM and EM use illumination to form larger and more detailed images of objects than the human eye can produce unaided. LM illuminates an object with photons of various sources of visible or infrared light in a natural setting, forming an image via the absorption and/or emission of light by the object. Meanwhile, EM illuminates the object with a beam of electrons emitted in a vacuum, forming the image via the scattering of electrons by the object. Electron microscopes are thus significantly more powerful than optical ones, but also more expensive, requiring painstaking sample preparation and, because of the need for a vacuum, applicable to non-living specimens only.
A glowing solution
Scientists determined to image living biospecimens on the smallest of scales have long been stymied by the limits of LM. That is, there’s only so much you can magnify a sample and still be able to preserve resolution - a law of nature referred to as Abbe’s Diffraction Limit, which sets the limit at 200nm when using natural light. This phenomenon has withstood human ingenuity until 20 years ago, when scientists Eric Betzig, William Moerner, and Stefan Hell (separately, in parallel) developed super-resolved fluorescence microscopy, for which they jointly received the 2014 Nobel Prize in Chemistry. In awarding the honor, the Royal Swedish Academy of Sciences said the trio “brought optical microscopy into the nanodimension.”
The three co-Laureates showed, in different ways, that it is possible to use so-called fluorophores—fluorescent chemicals that can absorb and then re-emit light when excited—to enhance resolution. For example, Stefan Hell and his colleagues developed stimulated emission depletion (STED) microscopy, which uses laser pulses to excite and then de-excite the fluorophores, producing a focal spot of light surrounded by a donut of darkness. The precision and power of the laser beams combine to break Abbe’s Diffraction Limit.
“It is an exciting time to be involved in the field of microscopy,” says Dr. Tali Dadosh, a staff scientist in the Weizmann Institute’s Electron Microscopy Unit. She specializes in super-resolution fluorescence LM, and in particular, stochastic optical reconstruction microscopy (STORM). The STORM method is based on randomly glowing fluorescent molecules which are used to label the sample (picture different colors for components of a cell). Unlike the precise glow of STED, in the STORM process the fluorescent dyes blink on and off at a rate that can be controlled by the chemical environment of the sample, so that the glow can be tuned.
Through a careful process of “glow-tuning”, Dr. Dadosh is able to explore the fine details of cells with nano-level precision. Dr. Dadosh helps Institute scientists design, conduct, and optimize a variety of exquisitely sensitive fluorescence microscopy experiments. She also makes use of a new technique that combines all these improvements in LM with the power of EM which offers a unique, high-resolution method for visualizing structural information about aspects of a cell.
Resolution evolution
Other forms of LM have been experiencing a steady and remarkable evolution. One of them is lightsheet microscopy, which allows for imaging intact organs, organisms, and embryos in 3D. It is also faster and more efficient than many other imaging techniques and gentler on biospecimens, causing far less photo (light) damage. The Institute plans to expand its capacity in LM in order to enable its scientists to conduct their research at the highest levels possible.
“Because of advances in light microscopy, scientists started to re-visit lightsheet microscopy, and now it is considered a relevant and highly valuable technology for biological sciences,” says Dr. Yoseph Addadi, who heads the de Picciotto-Lesser Cancer Cell Observatory in Memory of Wolfgang and Ruth Lesser (in the Moross Integrated Cancer Center, or MICC). He assists a range of Weizmann Institute life scientists with this technique, one of the newest technologies available in the MICC’s dedicated microscopy unit, thanks to the generosity of the Henry Krenter Foundation. In the past, this technology was used to image non-biological samples. But now, scientists can use it to follow the behavior of cancerous cells in live tissues for prolonged periods. Because it can penetrate deeper into tissues without damping fluorescent signals, scientists can literally watch the interactions between different cell populations in tumors.
Meanwhile, two-photon excitation microscopy, allows deep, live imaging of up to a 1mm thickness, and is based in infrared excitation of fluorescent dyes. This method results in a miniscule amount of excitation, in contrast to confocal or wide-field microscopes, and therefore prevents bleaching or other photo-damage to the tissue.
A number of Weizmann Institute scientists are using the extraordinary scanning sensitivity of two-photon microscopy, including scientists associated with the Krenter Institute, the MICC and beyond. For example, Dr. Ruth Scherz-Shouval of the Department of Biomolecular Sciences studies cancer cells and the way in which they recruit and subvert normal cells to create an environment that promotes tumor progression and metastasis. She uses two-photon microscopy to detect and visualize fine differences in the extracellular environment as a result of cancer progression.
Prof. Neeman developed a method for fluorescently labeling a type of cell normally involved in forming connective tissue—and which is frequently hijacked by ovarian cancer tumors to become part of the malignancy. These fibroblasts are thereby used as a beacon in two-photon microscopy, pointing to the otherwise invisible metastases, like an informant wearing a wire to a meeting with the mafia. This method not only finds hidden cancer cells, but it also enables the researchers to target delivery of antitumor therapies with specificity and sensitivity.
Pushing technology forward
As microscopy is already helping elucidate a panoply of important investigations, other scientists at the Weizmann Institute are forging ahead in the physics of it all, which undoubtedly will lead to further improvement in resolution.
For one, pushing past Abbe’s Diffraction Limit is an ongoing goal. Prof. Dan Oron, who heads the Department of Physics of Complex Systems, is exploring new approaches to simplify current microscopy techniques that can transcend the limit, and is applying these techniques to study biological systems. Prof. Nir Davidson, Dean of the Faculty of Physics, is an expert on laser physics and ultra-cold atomic physics who is hard at work focusing and shaping diffuse light beams, to this end.
Other scientists are trying to better understand how light behaves at very high intensities. Prof. Yaron Silberberg is a leader in laser technology, also referred to as nonlinear optics. His team has developed an approach that utilizes nonlinear optics in microscopy to uncover details about the behavior and dynamics of molecules. His approach may be particularly useful for learning about the dynamics of large molecules found within biological systems, revealing subtle, dynamic changes in a molecule’s structure, which affects how a molecule will interact with other molecules in its environment—e.g., the particular way in which a protein is folded determines its function in the human body.
Moskowitz Center makes (light) waves in imaging
Meanwhile, as LM is going nano, EM is going subnano. The revolution in EM involves single particles, stemming from transmission electron microscopy (TEM). The Irving and Cherna Moskowitz Center for Nano and Bio-Imaging, established in 2006 by Prof. Avi Minsky and now headed by Prof. Michael Elbaum, supports research in the Electron Microscopy Unit. Over the past year, the Unit has seen the integration of ultrahigh resolution light microscopy and electron microscopy. The Center helped purchase major equipment for one of the scanning EM microscopes, and will support the installation of a new camera for the TEM that allows determination of the structures of biomolecules at superb resolution.
In recent years, more than 30 publications by a wide range of Institute research groups used microscopy supported by the Moskowitz Center (and thus cite the center). Such investigations include those done by Prof. Reshef Tenne on new materials; Prof. Ernesto Joselevich on growing nanowires for electronics; Prof. Boris Rybtchinski on developing nanowires; Prof. Assaf Vardi on marine photosynthetic microorganisms; Prof. Lia Addadi on the formation of cholesterol crystals in atherosclerosis; and Prof. Avi Minsky on the replication cycles of giant viruses.
In TEM, the microscope emits an electron beam that interacts with the sample as it passes through the entire (very thin) sample. It can “see” objects with varying internal structures and the resulting image is a 2D projection of the sample. But while TEM, like other forms of EM, is a great method for obtaining atomic-resolution of inorganic materials, it is a killer on biospecimens. How then can scientists resolve protein structure, and thereby gain an understanding of protein function?
The most popular solution to this problem has been to use X-ray crystallography, in which the sample is crystallized and the protein structure is determined from a diffraction pattern. This technique is largely successful for analyses of biospecimens, but not for all proteins. That is, many proteins are very difficult to crystallize and researchers may spend years to find adequate crystallization conditions, so the process becomes a significant bottleneck. Consider that Prof. Yonath made a staggering 25,000 attempts before she succeeded in creating the first ribosome crystals.
So how can scientists obtain the extraordinary resolution of EM with recalcitrant proteins, without frying their samples to a crisp in the process? The answer today is a revolutionary technique of single-particle analysis conducted at cryogenic temperatures called single-particle cryo-EM.
In single-particle cryo-EM, a sample (e.g., a purified protein) is spread out sparsely and evenly on a grid, which is then plunged into a cryogenic liquid and flash-frozen—a process that preserves the sample’s natural structure. Next, the scientist uses an electron microscope to obtain multiple 2D images of each of the sparsely spread protein particles (hence the name ‘single-particle’), after which these images are used to construct a 3D model of the protein. Refinement of the 3D model to high resolution involves elaborate and intense computing. In this way, single-particle cryo-EM provides atomic and near-atomic imaging of viruses, ribosomes, ion channels, and various enzyme complexes.
The freezing process helps scientists contend with the unavoidable damage that all EM techniques cause to samples because the low temperatures protect sample integrity for the duration of the experiment. Nevertheless, no sample can withstand an electron beam’s intensity forever, and therefore the beam must be of low strength, administered bit by bit, so that the final 3D image is normally an average of tens of thousands of individual particles collected at low dose conditions.
Dr. Nadav Elad, an EM expert and colleague of Dr. Dadosh in the Electron Microscopy Unit, observes that it is the convergence of this technique with advances in technology that is the real revolution.
“People have been solving protein structure for decades,” he says. “But now, with improvements in electron microscopes, detectors, computer hardware and software, we are finally able to achieve atomic resolution of protein structure, making single-particle cryo-EM a valid alternative to X-ray crystallography.” True, there may be no avoiding the damaging effect of the electron beam completely. But scientists are hard at work to push the resolution boundaries even further, and there is much more work to be done in EM to advance capabilities.
Prof. Nir Davidson is funded by The Norman E. Alexander Family Foundation, The Center for Experimental Physics which he heads, The Deloro Institute for Advanced Research in Space and Optics which he heads, The Mary and Tom Beck - Canadian Center for Alternative Energy Research, Paul and Tina Gardner, Austin, TX, Leona M. and Harry B. Helmsley Charitable Trust, Dana and Yossie Hollander, Israel, Irving I. Moskowitz Foundation, Park Avenue Charitable Fund. Prof. Davidson is the incumbent of the Peter and Carola Kleeman Professorial Chair of Optical Sciences.
http://www.weizmann.ac.il/complex/NirDavidson
Prof. Michal Neeman heads the following centers: The Henry Chanoch Krenter Institute for Biomedical Imaging and Genomics; Clore Center for Biological Physics; Crown Human Genome Center; Dolfi and Lola Ebner Center for Biomedical Research; J & R Center for Scientific Research; The Willner Family Leadership Institute for the Weizmann Institute of Science; and the Yeda-Sela Center for Basic Research. She is supported by the Leona M. and Harry B. Helmsley Charitable Trust, Foundation Adelis, the Dukler Fund for Cancer Research, the Judy and Monroe Milstein Fund for Ovarian Cancer Research, the David M. Polen Charitable Trust, Andrew Adelson, Canada, and the European Research Council. Prof. Neeman is the incumbent of the Helen and Morris Mauerberger Professorial Chair in Biological Sciences.
http://www.weizmann.ac.il/Biological_Regulation/neeman
Prof. Dan Oron is funded by The Estate of Olga Klein Astrachan, The Mary and Tom Beck- Canadian Center for Alternative Energy Research, The Deloro Institute for Advanced Research in Space and Optics, European Research Council, Leona M. and Harry B. Helmsley Charitable Trust, Dana and Yossie Hollander, Israel, Willner Family Leadership Institute for the Weizmann Institute of Science, The Wolfson Family Charitable Trust.
http://www.weizmann.ac.il/complex/DOron
Dr. Ruth Scherz-Shouval is funded by The Estate of Annice Anzelewitz, Comisaroff Family Trust, Elsie and Marvin Dekelboum Family Foundation, Peter and Patricia Gruber Awards, Joseph Gurwin Foundation, The Laura Gurwin Flug Family Fund, Rising Tide Foundation, Estate of Mordecai M. Roshwald, Dr. Zwi & Amelia Steiger Cancer Research Fund. Dr. Scherz-Shouval is the incumbent of the Ernst and Kaethe Ascher Career Development Chair in Life Sciences.
http://www.weizmann.ac.il/Biomolecular_Sciences/Shouval
Prof. Yaron Silberberg is funded by The Crown Photonics Center which he heads, European Research Council, Wolfson Family Charitable Trust. Prof. Silberberg is the incumbent of the Harry Weinrebe Professorial Chair of Laser Physics.
http://www.weizmann.ac.il/complex/silberberg
Prof. Ben-Zion Shilo is funded by M.D. Moross Institute for Cancer Research, Carolito Stiftung, The Mary Ralph Designated Philanthropic Fund. Prof. Shilo is the incumbent of the Hilda and Cecil Lewis Professorial Chair of Molecular Genetics.
http://shilolabweb.weizmann.ac.il
Prof. Addadi is funded The Irving and Cherna Moskowitz Center for Nano and Nano - Bio Research, Jeanne and Joseph Nissim Foundation for Life Sciences Research. Prof. Addadi is the incumbent of the Dorothy and Patrick Gorman Professorial Chair.
http://www.weizmann.ac.il/Structural_Biology/Addadi
Prof. Ernesto Joselevich is funded by The Helen and Martin Kimmel Center for Nanoscale Science which he heads, European Research Council, The Irving and Cherna Moskowitz Center for Nano and Nano-Bio Research. Prof. Joselevich is the incumbent of the Drake Family Professorial Chair in Nanotechnology.
http://www.weizmann.ac.il/materials/ernesto
Prof. Abraham Minsky is funde by The Henry Krenter Institute for Biomedical Imaging and Genomics, The Irving and Cherna Moskowitz Center for Nano and Bio Nano Imaging. Prof. Minsky is the incumbent of the Professor T. Reichstein Professorial Chair.
http://www.weizmann.ac.il/Structural_Biology/minsky
Prof. Boris Rybtchinski is funded by Solo Dwek and Maurizio Dwek Research School of Chemical Science, Leona M. and Harry B. Helmsley Charitable Trust, Dana and Yossie Hollander, The Irving and Cherna Moskowitz Center for Nano and Bio-Imaging.
https://www.weizmann.ac.il/Organic_Chemistry/Rybtchinski
Prof. Reshef Tenne is funded by European Research Council, The Irving and Cherna Moscowitz Center for Nano and Bio-Imaging, Irving and Azelle Waltcher Endowed Research Fund in honor of Prof. Moshe Levy.
http://www.weizmann.ac.il/materials/tenne
Dr. Assaf Vardi is funded by The Mary and Tom Beck- Canadian Center for Alternative Energy Research, The Benoziyo Fund for the Advancement of Science, Brazil-Israel Energy Fund, The de Botton Center for Marine Science, Angel Faivovich Foundation for Ecological Research, Dana and Yossie Hollander, Israel, Scott Eric Jordan, The Irving and Cherna Moskowitz Center for Nano and Bio-Imaging, Rothschild Caesarea Foundation, David and Fela Shapell Family Foundation INCPM Fund for Preclinical Studies.
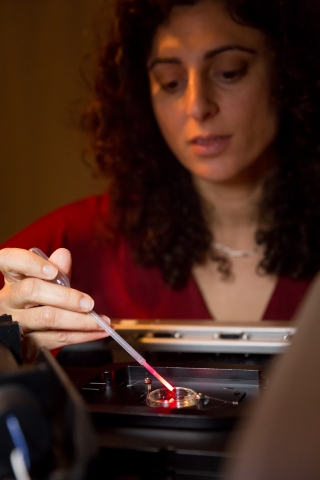
Dr. Tali Dadosh
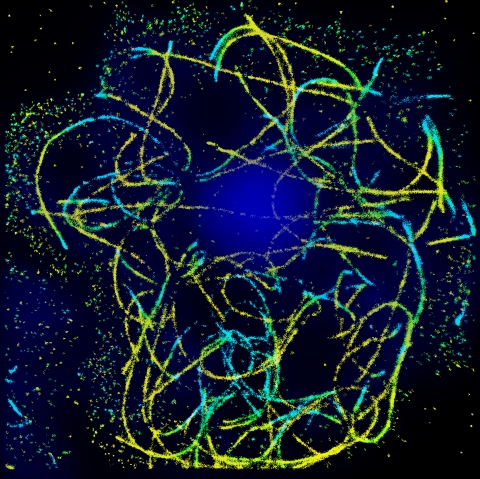
STORM imaging of microtubules in amoebae, courtesy of Dr. Tali Dadosh, as part of the studies conducted by Liran Ben Yaacov in the lab of Prof. Avi Minsky
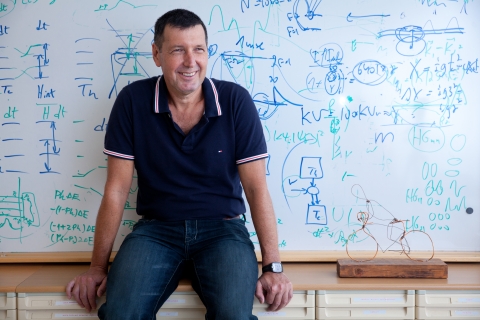
Prof. Nir Davidson

Derived from electron tomography, this image shows a modified vertex of the Mimivirus, one of the largest viruses ever discovered. The opening of this vertex, coined the “stargate”, generates a large portal through which the huge DNA genome of the virus is released into the host cytoplasm. Image taken with high-pressure freezing TEM, by Dr. Nathan Zauberman for his research on giant viruses in the lab of Prof. Avi Minsky of the Department of Structural Biology.