When an electron in an atom is excited by a photon, it typically decays back to the ground state. This “closed-cycle” allows for continuous photon scattering, and it is the basis for laser cooling and state detection in atomic systems.
When an electron in a molecule is excited by a photon, it decays back to the ground state while creating a random vibrational motion in the molecule. This is due to the finite overlap between the vibrational wavefunctions in the electronic excited and ground states (Franck-Condon factors). Thus, after scattering photons (from few in most molecules to hundreds in exceptional ones), the molecule will be “lost” in a highly excited vibrational state. Since molecules have no “closed-cycle” transitions, it is extremely difficult to cool their motion and detect their quantum state directly.
A method was developed in the trapped-ions community to overcome this problem - quantum logic. In quantum logic, a single atomic ion is co-trapped together with a single molecular ion in a radio-frequency ion trap (see figure). The two ions align where the forces originate from their Coulomb repulsion and the trap’s confinement balance. Similar to strongly coupled pendulums, the two ions share normal modes of motion. This shared motion allows cooling, controlling, and detecting the molecule via the atomic ion.
Quantum-logic protocols have a widespread application in the trapped-ions community. Apart from molecular systems, they are also used in quantum computers, optical atomic clocks, and experiments with highly-charged ions.
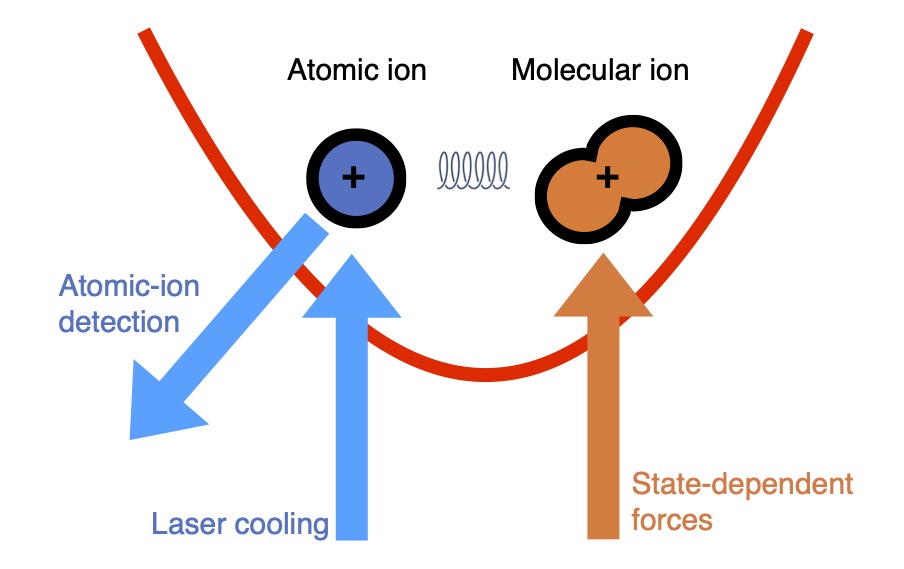
Quantum logic. Atomic and molecular ions are trapped in a harmonic potential and are coupled by their mutual Coulomb interaction. Laser cooling on the atomic ion dissipates the energy of the shared motion of the two-ion crystal. State-dependent forces couple the state of the molecular ion with that of the shared motion, which is then detected via the atomic ion.