Super Resolution in Ultrasound and Microscopy
The attainable resolution of ultrasonography and optical imaging is fundamentally limited by wave diffraction, i.e., the minimum distance between separable scatters is half a wavelength. Different biomedical applications can benefit from an increased resolution that will enable the recovery and visualization of small structures with sub-diffraction resolution. For example, small blood vessels (< 100 um) and organelles within biological cells (< 100 nm).
In our lab, we aim to achieve super resolution by utilizing a Nobel prize-winning concept that takes advantage of a series of frames, each composed of a sparse distribution of emitters. The main assumption in this method is that the emitters in each frame are resolvable and therefore the location of each of them is estimated with sub-diffraction resolution. In our lab, we further improve this idea by applying advanced, model-based learning methods for signal processing and optimization, which enable us to super resolve the overall structure of interest using a small number of frames and in real-time without requiring the data to be resolvable. This paves the way to optical imaging of dynamic cellular processes at a molecular scale, and deep-tissue ultrasound imaging for intricate ultrasonography tasks like micro-vascular imaging.
Our methods can learn even from a single image, can be implemented efficiently in real-time, and pave the way to live cell imaging.
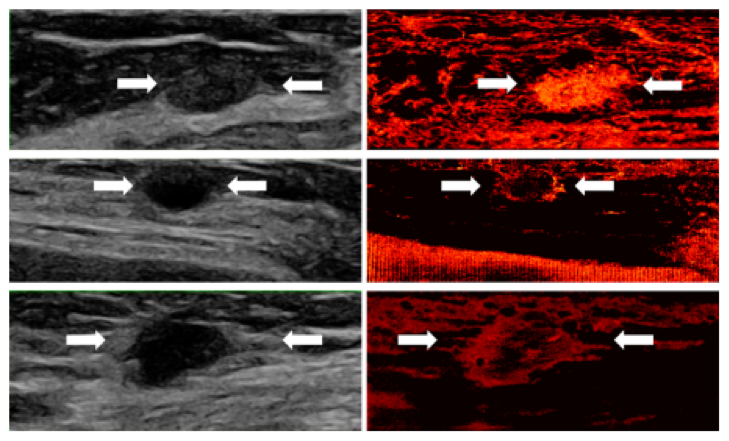

References
- O. Bar-Shira, A. Grubstein, Y. Rapson, D. Suhami, E. Atar, K. Peri-Hanania, R. Rosen, Y. C. Eldar, "Learned Super Resolution Ultrasound for Improved Breast Lesion Characterization", 24th International Conference on Medical Image Computing and Computer Assisted Intervention (MICCAI 2021), October 2021.
- G. Dardikman-Yoffe and Y. C. Eldar, "Learned SPARCOM: Unfolded Deep Super-Resolution Microscopy", Optics Express, vol. 28, issue 19, pp. 27736-27763, September 2020.
- A. Bar-Zion, O. Solomon, C. Tremblay-Darveau, D. Adam and Y. C. Eldar, "SUSHI: Sparsity-Based Ultrasound Super-Resolution Hemodynamic Imaging", IEEE Transactions on Ultrasonics, Ferroelectrics and Frequency Control, vol. 65, issue 12, pp. 2365-2380, December 2018.
- O. Solomon, R. J. G. van Sloun, H. Wijkstra, M. Mischi and Y. C. Eldar, "Exploiting Flow Dynamics for Super-Resolution in Contrast-Enhanced Ultrasound", IEEE Transactions on Ultrasonics, Ferroelectrics, and Frequency Control, vol. 66, issue 10, pp. 1573-1586, October 2019.
-
R. J. G. van Sloun, O. Solomon, M. Bruce, Z. Z. Khaing, H. Wijkstra, Y. C. Eldar and M. Mischi, "Super-Resolution Ultrasound Localization Microscopy through Deep Learning", IEEE Transactions on Medical Imaging, vol. 40, issue 3, pp. 829-839, March 2021.